Startup Overview#
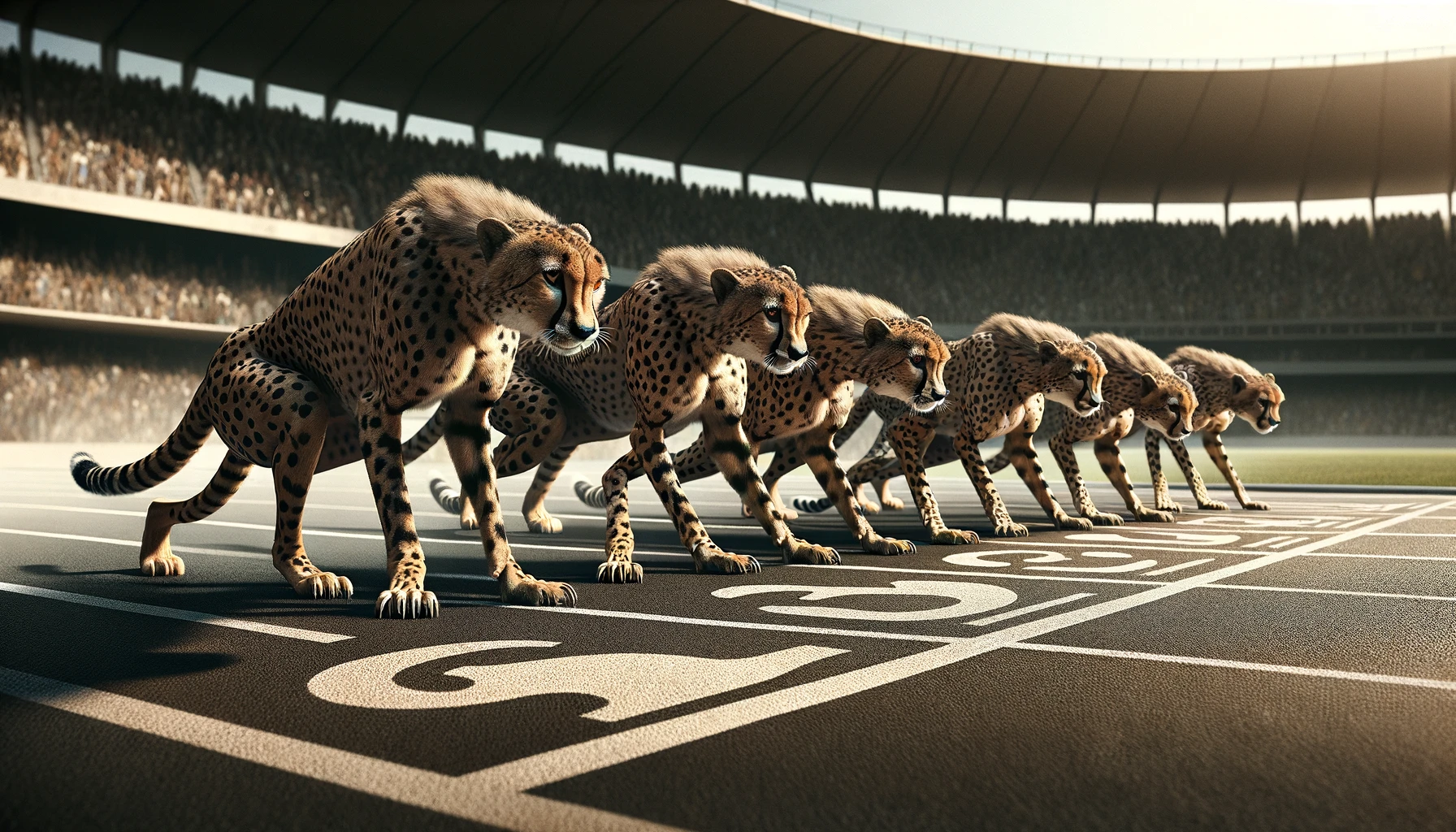
When referring to startup, starts or restarts, we are referring to the startup of the data nodes. To start up a cluster, one must first however start up a management server, which contains the cluster configuration.
In the following we describe the different types of data node startups, how they can be configured, what they run and how they can be monitored.
Startup Types#
RonDB defines the following four types of startup:
-
Initial Cluster Start
-
Cluster Restart - cluster failed / was shut down
-
Node Restart - cluster stays operational
-
Initial Node Restart - cluster stays operational
The difference between a node restart and an initial node restart is
that the latter starts with an empty file system. Any initial (re-)start
is also signalled executing the data node binary as such:
ndbmtd --initial
.
Use-cases for node restarts:
-
Propagating configuration changes (rolling restarts)
-
OS upgrades
-
Simple software up-/downgrades
Use-cases for initial node restarts:
-
The node’s file system was corrupted
-
Moving node to a different machine / VM with local NVMe drives
-
Complex software up-/downgrades; Downgrades are needed for upgrade roll-backs
The described startup types will be used to better explain the startup process in upcoming sections.